TIRF is a widely used tool for effectively creating a super resolution instrument out of a standard widefield microscope. The cool part of TIRF is that it bypasses the limits of axial resolution on the scope not by altering the optics per-se, but by altering what gets excited. In a normal widefield fluorescent microscope, photons are emitted from an arc lamp, LED, or other source that are of numerous polarization, phase and angular states. In effect you shower the specimen by flooding light through the objective, exciting any fluorescent molecule in the path of the light. The problem is that what gets excited may be inside, below or out of the objective’s focal plane. Any image you collect from such a setup represents both the “signal” (molecules in focus) and “noise” (molecules that aren’t in focus) in the field of view. More importantly, It’s common to have out of focus noise overpower in-focus signal, thereby reducing or restricting what information can be obtained from the microscope. My fancy cartoon below shows an example of a widefield system and it’s illumination path.
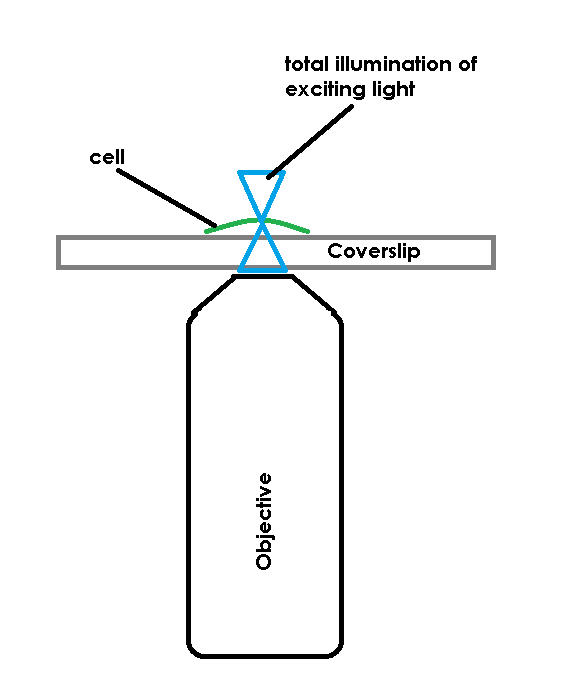
Of course, one simple solution, but an expensive one, to this problem is to add a pinhole-based confocal to the optical system. While a confocal does reduce or eliminate the out of focus haze in the image, it doesn’t provide any increase in Z accuracy. This axial resolution limit is still based on the objective’s performance.
TIRF avoids this limit by restricting the excitation field. In order to accomplish this, TIRF uses a laser, coupled to the microscope and most commonly delivered through the objective, to deliver excitation energy into the specimen. The trick is not that the laser excites the fluorescent molecules, the trick is that the laser, if set at a proper angle (critical angle) will bounce off of the bottom of a specimen container. When the laser bounces back there is a small electromagnetic field created, just on the specimen-side of the coverslip. This EM field is the same frequency as the bounced laser light, allowing it to excite fluorescent molecules. The field has an exponential decay as it extends from the coverslip, so it’s only strong enough to excite molecules that are very close to the interface of the coverslip and the specimen (usually the excitation field in TIRF is ~100nm of depth).
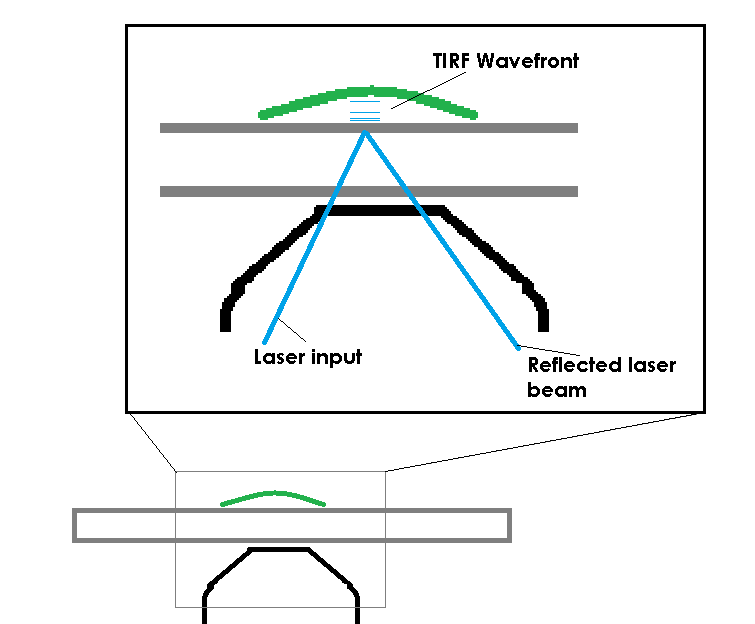
It’s hard to believe that light could be reflected back into a medium simply because of a refractive index change, but I was able to visually capture this effect on a large scale when installing a customer’s system at UC Davis. The image below is of a fluid filled jar, with some bits of glass tube floating in it. The cool part is the laser beam. You can see the beam emitting from the objective and passing through the fluid. When the beam reaches the air (lower refractive index), the refractive index change is at or beyond the critical angle the light can follow, so the light is reflected back into the previous, higher refractive index medium (the fluid). This is the fundamental effect that makes TIRF possible.
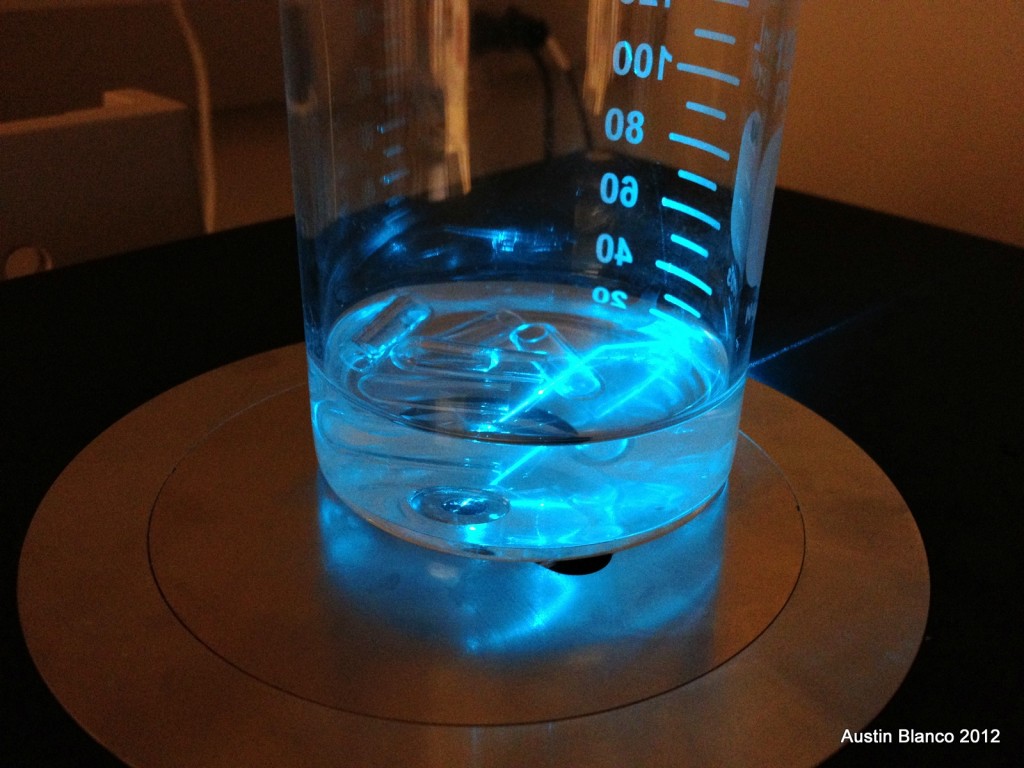
The limitations to this technique are that you need a laser, you need an optic that can reach the critical angle, and you need to have something in your research that can sit on the coverslip, i.e. this won’t work for anything farther than ~100nm from the coverslip. If everything lines up, you get an image representing roughly a 10x improvement in Z resolution over a confocal microscope, qualifying this as a “super resolution” technique.
If you’d like to learn more about the theory of TIRF microscopy I highly recommend the MicroscopyU explanation, which does a better job of explaining the physics than my overview here.
***Thanks to Professor Jawdat Al-Bassam of UC Davis for allowing me to demonstrate this effect on his instrument!
-Austin